Superhabitable planet

A superhabitable world is a hypothetical type of planet or moon that is better suited than Earth for the emergence and evolution of life. The concept was introduced in a 2014 paper by René Heller and John Armstrong, in which they criticized the language used in the search for habitable exoplanets and proposed clarifications.[2] The authors argued that knowing whether a world is located within the star's habitable zone is insufficient to determine its habitability, and that the prevailing model of characterization was geocentric or anthropocentric in nature. Instead, they proposed a biocentric model that prioritized characteristics affecting the abundance of life and biodiversity on a world's surface.[2]
If a world possesses more diverse flora and fauna than there are on Earth, then it would empirically show that its natural environment is more hospitable to life.[3] To identify such a world, one should consider its geological processes, formation age, atmospheric composition, ocean coverage, and the type of star that it orbits. In other words, a superhabitable world would likely be larger, warmer, and older than Earth, with an evenly-distributed ocean, and orbiting a K-type main-sequence star.[4] In 2020, astronomers, building on Heller and Armstrong's hypothesis, identified 24 potentially superhabitable exoplanets based on measured characteristics that fit these criteria.[5]
Stellar characteristics

A star's characteristics is a key consideration for planetary habitability.[6] The types of stars generally considered to be potential hosts for habitable worlds include F, G, K, and M-type main-sequence stars.[7] The most massive stars—O, B, and A-type, respectively—have average lifespans on the main sequence that are considered too short for complex life to develop,[8] ranging from a few hundred million years for A-type stars to only a few million years for O-type stars.[9] Thus, F-type stars are described as the "hot limit" for stars that can potentially support life, as their lifespan of 2 to 4 billion years would be sufficient for habitability.[10] However, F-type stars emit large amounts of ultraviolet radiation, and without the presence of a protective ozone layer, could disrupt nucleic acid-based life on a planet's surface.[10]
On the opposite end, the less massive red dwarfs, which generally includes M-type stars, are by far the most common and long-lived stars in the universe,[11] but ongoing research points to serious challenges to their ability to support life. Due to the low luminosity of red dwarfs, the circumstellar habitable zone (HZ)[a] is in very close proximity to the star, which causes any planet to become tidally locked.[14] The primary concern for researchers, however, is the star's propensity for frequent outbreaks of high-energy radiation, especially early in its life, that could strip away a planet's atmosphere.[15] At the same time, red dwarfs do not emit enough quiescent UV radiation (i.e., UV radiation emitted during inactive periods) to support biological processes like photosynthesis.[3]
Dismissing both ends, astronomers are led to conclude that G and K-type stars—yellow and orange dwarfs, respectively—provide the best life-supporting characteristics. However, a limiting factor to the habitability of yellow dwarfs is their higher emissions of ionizing radiation and shorter lifespans compared to cooler orange dwarfs.[16] Therefore, researchers conclude that orange dwarfs offer the best conditions for a superhabitable world.[3][16]
Also nicknamed "Goldilocks stars," orange dwarfs emit low enough levels of ultraviolet radiation to eliminate the need for a protective ozone layer, but just enough to contribute to necessary biological processes.[17][3] Additionally, the long average lifespan of an orange dwarf (18 to 34 billion years, compared to 10 billion for the Sun) provides a more stable habitable zone throughout the star's lifetime, providing more time for life to develop.[18][19][17]
Planetary characteristics
Age

It is necessary for the age of any superhabitable world to be greater than Earth's age (~4.5 billion years).[19] This necessity is based on the belief that as a planet or moon ages, it experiences increasing levels of biodiversity, since native species have had more time to evolve, adapt, and stabilize the environmental conditions suitable for life.[19] However, the eventual exhaustion of a world's internally generated heat means that there is also an upper limit to the age of any habitable world; internal cooling would lead to changes to the average global temperature and atmospheric composition.[20] Therefore, the optimal age range for a superhabitable world would be roughly 5–8 billion years.[20]
Orbital characteristics

During the main sequence phase, a star burns hydrogen in its core, producing energy through nuclear fusion. Over time, as the hydrogen fuel is consumed, the star's core contracts and heats up, leading to an increase in the rate of fusion. This causes the star to gradually become more luminous, and as its luminosity increases, the amount of energy it emits grows, pushing the habitable zone (HZ) outward.[23][24] Studies suggest that Earth's orbit lies near the inner edge of the Solar System's HZ,[14] which could harm its long-term livability as it nears the end of its HZ lifetime.
Ideally, the orbit of a superhabitable world should be further out and closer to the center of the HZ relative to Earth's orbit,[25][26] but knowing whether a world is in this region is insufficient on its own to determine habitability.[3] Not all rocky planets in the HZ may be habitable, while tidal heating can render planets or moons habitable beyond this region. For example, Jupiter's moon Europa is well beyond the outer limits of the Solar System's HZ, yet as a result of its orbital interactions with the other Galilean moons, it is believed to have a subsurface ocean of liquid water beneath its icy surface.[27]
According to a 2023 paper by Jonathan Jernigan and colleagues, marine biological activity increases on planets with increasing obliquity and eccentricity. The authors suggest that planets with a high obliquity and/or eccentricity may be superhabitable, and that scientists should be keen to look for biosignatures on exoplanets with these orbital characteristics.[28]
Mass and size

Assuming that a greater surface area would provide greater biodiversity, the size of a superhabitable world should generally be greater than 1 R🜨, with the condition that its mass is not arbitrarily large.[29] Studies of the mass-radius relationship indicate that there is a transition point between rocky planets and gaseous planets (i.e., mini-Neptunes) that occurs around 2 M🜨 or 1.7 R🜨.[30][31] Another study argues that there is a natural radius limit, set at 1.6 R🜨, below which nearly all planets are terrestrial, composed primarily of rock-iron-water mixtures.[32]
Heller and Armstrong argue that the optimal mass and radius of a superhabitable world can be determined by geological activity; the more massive a planetary body, the longer time it will continuously generate internal heat—a major contributing factor to plate tectonics.[29] Too much mass, however, can slow plate tectonics by increasing the pressure of the mantle.[29] It is believed that plate tectonics peak in bodies between 1 and 5 M🜨, and from this perspective, a planet can be considered superhabitable up to around 2 M🜨.[33] Assuming this planet has a density similar to Earth's, its radius should be between 1.2 and 1.3 R🜨.[33][29]
Geology

An important geological process is plate tectonics, which appears to be common in terrestrial planets with a significant rotation speed and an internal heat source.[34] If large bodies of water are present on a planet, plate tectonics can maintain high levels of carbon dioxide (CO
2) in its atmosphere and increase the global surface temperature through the greenhouse effect.[35] However, if tectonic activity is not significant enough to increase temperatures above the freezing point of water, the planet could experience a permanent ice age, unless the process is offset by another energy source like tidal heating or stellar irradiation.[36] On the other hand, if the effects of any of these processes are too strong, the amount of greenhouse gases in the atmosphere could cause a runaway greenhouse effect by trapping heat and preventing adequate cooling.
The presence of a magnetic field is important for the long-term survivability of life on the surface of a planet or moon.[22] A sufficiently strong magnetic field effectively shields a world's surface and atmosphere against ionizing radiation emanating from the interstellar medium and its host star.[22][37] A planet can generate an intrinsic magnetic field through a dynamo that involves an internal heat source, an electrically conductive fluid like molten iron, and a significant rotation speed, while a moon could be extrinsically protected by its host planet's magnetic field.[22] Less massive bodies and those that are tidally locked are likely to have a weak to non-existent magnetic field, which over time can result in the loss of a significant portion of its atmosphere by hydrodynamic escape and become a desert planet.[29] If a planet's rotation is too slow, such as with Venus, then it cannot generate an Earth-like magnetic field. A more massive planet could overcome this problem by hosting multiple moons, which through their combined gravitational effects, can boost the planet's magnetic field.[38]
Surface features
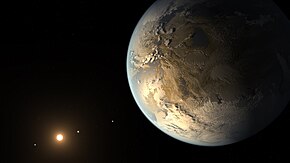
The appearance of a superhabitable world should be similar to the conditions found in the tropical climates of Earth.[39] Due to the denser atmosphere and less temperature variation across its surface, such a world would lack any major ice sheets and have a higher concentration of clouds, while plant life would potentially cover more of the planet's surface and be visible from space.[39]
When considering the differences in the peak wavelength of visible light for K-type stars and the lower stellar flux of the planet, surface vegetation may exhibit colors different than the typical green color found on Earth.[40][41] Instead, vegetation on these worlds could have a red, orange, or even purple appearance.[42]
An ocean that covers a large portion of a world's surface with fractionate continents and archipelagos could provide a stable environment across its surface.[43] In addition, the greater surface gravity of a superhabitable world could reduce the average ocean depth and create shallow ocean basins, providing the optimal environment for marine life to thrive.[44][45][46] For example, marine ecosystems found in the shallow areas of Earth's oceans and seas, given the amount of light and heat they receive, are observed to have greater biodiversity and are generally seen as being more comfortable for aquatic species. This has led researchers to speculate that shallow water environments on exoplanets should be similarly suitable for life.[43][47]
Climate
In general, the climate of a superhabitable planet would be warm, moist, and homogeneous, allowing life to extend across the surface without presenting large population differences.[48][49] These characteristics are in contrast to those found on Earth, which has more variable and inhospitable regions that include frigid tundra and dry deserts.[50] Deserts on superhabitable planets would be more limited in area and would likely support habitat-rich coastal environments.[51]
The optimum surface temperature for Earth-like life is unknown, although it appears that on Earth, organism diversity has been greater in warmer periods.[52] It is therefore possible that exoplanets with slightly higher average temperatures than that of Earth are more suitable for life.[53] The denser atmosphere of a superhabitable planet would naturally provide a greater average temperature and less variability of the global climate.[54][46] Ideally, the temperature should reach the optimal levels for plant life, which is 25 °C (77 °F). In addition, a large distributed ocean would have the ability to regulate a planet's surface temperature similar to Earth's ocean currents, and could allow it to maintain a moderate temperature within the habitable zone.[55][51]
There are no solid arguments to explain if Earth's atmosphere has the optimal composition,[56] but relative atmospheric oxygen levels is required to meet the high-energy demands of complex life (O
2).[57] Therefore, it is hypothesized that oxygen abundance in the atmosphere is essential for complex life on other worlds.[56][57]
List of potentially superhabitable exoplanets
In September 2020, Dirk Schulze-Makuch and colleagues identified 24 contenders for superhabitable planets out of more than 4000 confirmed exoplanets and exoplanet candidates.[5] The criteria included measurable factors like type of star, and the planet's age, mass, radius, and surface temperature. The authors also considered more hypothetical factors like the presence of abundant water, a large moon, and a geological recycling mechanism like plate tectonics.[20]
Kepler-1126b (KOI-2162.01) and Kepler-69c (KOI-172.02) are the only objects in the list that have been confirmed as exoplanets.[58] However, earlier research on Kepler-69c suggests that because its orbit lies near the inner edge of the HZ, its atmosphere could likely be in a runaway greenhouse state, which could heavily impact its prospects for habitability.[59] The full list can be found below.[60]
Object | Star type | Age (Gyr) | Mass (M⊕) | Radius (R⊕) | Surface temp. (K) | Period (days) | Distance (ly) | Refs/Notes |
---|---|---|---|---|---|---|---|---|
Earth | G2V | 4.54 | 1.00 | 1.00 | 287 | 365.25 | 0 | Reported for reference. Only planet known to support life.[61] |
KOI-5878.01 | K3V-IV | 8.0 | — | 1.88 | 522 | 211.54 | 10297 | Not to be confused with KOI-4878.01. |
KOI-5237.01 | G8.5IV | 7.0 | — | 1.84 | 429 | 380.39 | 9481 | |
KOI-7711.01 | G9V-IV | 7.5 | — | 1.31 | 413 | 302.78 | 4416 | |
Kepler-1126b | G1V | 7.5 | 3.64 | 1.73 | 401 | 108.59 | 2114 | Confirmed exoplanet.[62] |
Kepler-69c | G4V | 7.0 | 3.57 | 1.71 | 356 | 242.47 | 2433 | Confirmed exoplanet. Likely a super-Venus.[63][64] |
KOI-5248.01 | G8.5V | 4.3 | — | 1.35 | 355 | 179.25 | 3640 | |
KOI-5176.01 | G8.5V | 2.55 | — | 1.26 | 335 | 215.73 | 1611 | |
KOI-7235.01 | G9V | 6.5 | — | 1.15 | 329 | 299.67 | 3712 | |
KOI-7223.01 | G3V | 5.5 | — | 1.59 | 312 | 317.05 | 3389 | |
KOI-7621.01 | G3V | 0.82 | — | 1.86 | 308 | 275.07 | 365 | |
KOI-5135.01 | K2.5V | 5.9 | — | 2 | 304 | 314.77 | 4247 | |
KOI-5819.01 | K0V | 4.3 | — | 1.24 | 300 | 381.38 | 2701 | |
KOI-5554.01 | G1.5V | 6.5 | — | 0.96 | 299 | 362.22 | 701 | |
KOI-7894.01 | G4V | 5.0 | — | 1.62 | 289 | 347.98 | 3053 | |
KOI-456.04 | G8V | 7.0 | — | 1.91 | 287 | 378.42 | 3141 | Orbits a sun-like star with an Earth-like orbital period.[65] |
KOI-5715.01 | K3V | 5.5 | — | 1.93 | 285 | 189.96 | 2965 | One of the more promising candidates for superhabitability.[60] |
KOI-5276.01 | K2.8V | 6.0 | — | 2 | 280 | 220.72 | 3249 | |
KOI-8000.01 | G8V | 7.5 | — | 1.7 | 279 | 225.49 | 2952 | |
KOI-8242.01 | G7V | 5.5 | — | 1.36 | 270 | 331.56 | 1963 | |
KOI-5389.01 | K1.5V | 2.9 | — | 1.73 | 293 | 365.74 | 1018 | |
KOI-5130.01 | K2.5V | 6.0 | — | 1.9 | 305 | 370.06 | 3076 | |
KOI-5978.01 | K3V | — | — | 1.78 | 238 | 364.20 | — | |
KOI-8047.01 | K3V | 0.76 | — | 1.98 | 226 | 302.34 | 1924 |
See also
Notes
- ^ The habitable zone (HZ) is a region present around each star where a terrestrial planet or moon, given the right physical conditions, could maintain liquid water on its surface.[12][13]
- ^ The initials "HZD" or "Habitable Zone Distance" mark the position of a planet about the center of the habitable zone of the system (value 0). A negative HZD value means that the orbit of a planet is smaller near its star —the center of the habitable zone— while a positive value means a wider orbit around its star. The values 1 and −1 mark the boundary of the habitable zone.[21] A superhabitable planet should have a HZD of 0 (the optimal location within the habitable zone).[22]
References
- ^ Kiang 2008, pp. 48–55.
- ^ a b Heller & Armstrong 2014, p. 50.
- ^ a b c d e Heller & Armstrong 2014, p. 57.
- ^ Heller & Armstrong 2014, p. 55-59.
- ^ a b Schulze-Makuch, Heller & Guinan 2020, p. 1394.
- ^ Tuchow & Wright 2022.
- ^ Palma n.d.
- ^ Weidner & Vink 2010.
- ^ Jones 2016.
- ^ a b Sato et al. 2014.
- ^ Laughlin, Bodenheimer & Adams 1997.
- ^ Kopparapu et al. 2013, p. 2.
- ^ Huang 1959, p. 397.
- ^ a b Kopparapu et al. 2013.
- ^ France et al. 2020.
- ^ a b Schulze-Makuch, Heller & Guinan 2020, p. 1396.
- ^ a b Cuntz & Guinan 2016.
- ^ Rushby et al. 2013.
- ^ a b c Heller & Armstrong 2014, p. 56-57.
- ^ a b c Schulze-Makuch, Heller & Guinan 2020, p. 1398.
- ^ Méndez 2011.
- ^ a b c d Heller & Armstrong 2014, p. 56.
- ^ Parker, Charles Thomas; Garrity, George M (18 April 2013). Parker, Charles Thomas; Garrity, George M. (eds.). "Exemplar Abstract for Marinivirga aestuarii Park et al. 2013, Algibacter aestuarii (Park et al. 2013) Park et al. 2013 and Hyunsoonleella aestuarii (Park et al. 2013) Li et al. 2022". The NamesforLife Abstracts. doi:10.1601/ex.24037. Retrieved 12 September 2024.
- ^ Sahé, Kaavje; insightshub.in (12 September 2024). "Can we extend Earth's habitability?". InsightsHub. Archived from the original on 12 September 2024. Retrieved 12 September 2024.
- ^ Mendéz 2012.
- ^ Heller & Armstrong 2014, pp. 56, 58.
- ^ Reynolds, McKay & Kasting 1987.
- ^ Jernigan et al. 2023, p. 7-10.
- ^ a b c d e Heller & Armstrong 2014, p. 55.
- ^ Chen & Kipping 2016.
- ^ Buchhave et al. 2014.
- ^ Rogers 2015.
- ^ a b Noack & Breuer 2011.
- ^ Riguzzi et al. 2010.
- ^ Van Der Meer et al. 2014.
- ^ Walker, Hays & Kasting 1981.
- ^ Baumstark-Khan & Facius 2002.
- ^ Heller & Armstrong 2014, pp. 57–58.
- ^ a b Heller & Armstrong 2014, pp. 54–59.
- ^ Kiang 2008.
- ^ Than 2007.
- ^ Skibba 2023.
- ^ a b Heller & Armstrong 2014, p. 54.
- ^ Cowan & Abbot 2014.
- ^ Heller & Armstrong 2014, p. 54-56.
- ^ a b Pierrehumbert 2010.
- ^ Gray 1997.
- ^ Heller & Armstrong 2014, pp. 55–58
- ^ Moyer 2014.
- ^ Moeller 2013.
- ^ a b Heller & Armstrong 2014, pp. 55–56.
- ^ Mayhew et al. 2012.
- ^ Heller & Armstrong 2014, p. 55-56.
- ^ Heller & Armstrong 2014, p. 59.
- ^ Cullum, Stevens & Joshi 2014.
- ^ a b Heller & Armstrong 2014, p. 58.
- ^ a b Catling et al. 2005, p. 415.
- ^ Schulze-Makuch, Heller & Guinan 2020, p. 1399.
- ^ Kane, Barclay & Gelino 2015, pp. 4–5.
- ^ a b Schulze-Makuch, Heller & Guinan 2020, p. 1401.
- ^ Greshko n.d.
- ^ Morton et al. 2016.
- ^ Barclay et al. 2013.
- ^ Kane, Barclay & Gelino 2015.
- ^ Heller & Krummheuer 2020.
Sources
- Barclay, Thomas; Burke, Christopher J.; Howell, Steve B.; Rowe, Jason F.; Huber, Daniel; Isaacson, Howard; Jenkins, Jon M.; Kolbl, Rea; Marcy, Geoffrey W.; Quintana, Elisa V. (18 April 2013). "A Super-Earth-sized Planet Orbiting in or Near the Habitable Zone around a Sun-like Star". The Astrophysical Journal. 768 (2): 101. arXiv:1304.4941. Bibcode:2013ApJ...768..101B. doi:10.1088/0004-637X/768/2/101.
- Baumstark-Khan, Christa; Facius, Rainer (January 2002). "Life under Conditions of Ionizing Radiation". Astrobiology - The Quest for the Conditions of Life. pp. 261–284. doi:10.1007/978-3-642-59381-9_18. ISBN 978-3-642-63957-9.
- Buchhave, Lars A.; Bizzarro, Martin; Latham, David W.; Sasselov, Dimitar; Cochran, William D.; Endl, Michael; Isaacson, Howard; Juncher, Diana; Marcy, Geoffrey W. (28 May 2014). "Three regimes of extrasolar planets inferred from host star metallicities". Nature. 509 (7502): 593–595. arXiv:1405.7695. doi:10.1038/nature13254. PMC 4048851. PMID 24870544.
- Catling, David C.; Glein, Christopher R.; Zahnle, Kevin J.; McKay, Christopher P. (7 June 2005). "Why O2 is required by complex life on habitable planets and the concept of planetary 'oxygenation time'" (PDF). Astrobiology. 5 (3): 415–438. doi:10.1089/ast.2005.5.415. PMID 15941384. Archived (PDF) from the original on 8 February 2024. Retrieved 8 February 2024.
- Chen, Jingjing; Kipping, David (2016). "Probabilistic Forecasting of the Masses and Radii of Other Worlds". The Astrophysical Journal. 834 (1): 17. arXiv:1603.08614. doi:10.3847/1538-4357/834/1/17. S2CID 119114880.
- Cooper, Keith (4 September 2012). "When Did the Universe Have the Right Stuff for Planets?". Space.com.
- Gray, John S. (1997). "Marine biodiversity: patterns, threats, and conservation needs". Biodiversity & Conservation. 6 (6): 153–175. doi:10.1023/A:1018335901847. S2CID 11024443.
- Harrison, J.F.; Kaiser, A.; VandenBrooks, J.M. (26 May 2010). "Atmospheric oxygen level and the evolution of insect body size". Proceedings of the Royal Society B. 277 (1690): 1937–1946. doi:10.1098/rspb.2010.0001. PMC 2880098. PMID 20219733.
- Heller, René; Krummheuer, Birgit (4 June 2020). "A faint resemblance of Sun and Earth". Max-Planck-Gesellschaft. Archived from the original on 8 February 2024. Retrieved 6 February 2024.
- Heller, René; Armstrong, John (2014). "Superhabitable Worlds". Astrobiology. 14 (1): 50–66. arXiv:1401.2392. Bibcode:2014AsBio..14...50H. doi:10.1089/ast.2013.1088. PMID 24380533.
- Huang, Su-Shu (September 1959). "Occurrence of Life in the Universe". American Scientist. Vol. 47, no. 3. pp. 397–402. JSTOR 27827376.
- Jernigan, Jonathan; Laflèche, Émilie; Burke, Angela; Olson, Stephanie (2023). "Superhabitability of High-obliquity and High-eccentricity Planets". Astrophysical Journal. 944 (2): 205. arXiv:2303.02188v1. Bibcode:2023ApJ...944..205J. doi:10.3847/1538-4357/acb81c.
- Jones, Jeremy W. (12 August 2016). The Ages of A-Stars (PhD Dissertation). Georgia State University. doi:10.57709/8869793. Archived from the original on 7 February 2024. Retrieved 7 February 2024.
- Kiang, Nancy A. (April 2008). "The color of plants on other worlds". Scientific American. Vol. 298, no. 4. pp. 48–55. Bibcode:2008SciAm.298d..48K. doi:10.1038/scientificamerican0408-48. Archived from the original on 8 February 2024.
- Kivelson, Margaret Galland; Bagenal, Fran (2014). Encyclopedia of the Solar System (3rd ed.). Elsevier Inc. pp. 137–157. doi:10.1016/B978-0-12-415845-0.00007-4. ISBN 978-0-12-415845-0.
- Kopparapu, R.K.; Ramirez, R.; Kasting, J.; Eymet, V. (2013). "Habitable Zones around Main-Sequence Stars: New Estimates". Astrophysical Journal. 765 (2): 131. arXiv:1301.6674. Bibcode:2013ApJ...765..131K. doi:10.1088/0004-637X/765/2/131.
- Laughlin, G.; Bodenheimer, P.; Adams, F. C. (1997). "The End of the Main Sequence". The Astrophysical Journal. 482 (1): 420–432. Bibcode:1997ApJ...482..420L. doi:10.1086/304125.
- Cowan, Nicolas B.; Abbot, Dorian S. (2 January 2014). "Water Cycling between Ocean and Mantle: Super-Earths Need Not Be Waterworlds". The Astrophysical Journal. 781 (27): 27. arXiv:1401.0720. Bibcode:2014ApJ...781...27C. doi:10.1088/0004-637X/781/1/27. S2CID 56272100.
- Mayhew, P.J.; Bell, M.A.; Benton, T.G.; McGowan, A.J. (2012). "Biodiversity tracks temperature over time". Proceedings of the National Academy of Sciences. 109 (38): 15141–15145. Bibcode:2012PNAS..10915141M. doi:10.1073/pnas.1200844109. PMC 3458383. PMID 22949697.
- Moeller, Karla (19 July 2013). "Boundless Biomes". ASU - Ask A Biologist. Archived from the original on 6 February 2024. Retrieved 6 February 2024.
- Méndez, Abel (10 August 2011). "Habitable Zone Distance (HZD): A habitability metric for exoplanets". PHL. Archived from the original on 6 July 2015. Retrieved 22 July 2015.
- Mendéz, Abel (30 June 2012). "Habitable Zone Atmosphere (HZA): A habitability metric for exoplanets". Planetary Habitability Laboratory. Archived from the original on 15 July 2015. Retrieved 16 July 2015.
- Morton, Timothy D.; Bryson, Stephen T.; Coughlin, Jeffrey L.; Rowe, Jason F.; Ravichandran, Ganesh; Petigura, Erik A.; Haas, Michael R.; Batalha, Natalie M. (May 2016). "False Positive Probabilities for all Kepler Objects of Interest: 1284 Newly Validated Planets and 428 Likely False Positives". The Astrophysical Journal. 822 (2): 86. arXiv:1605.02825. Bibcode:2016ApJ...822...86M. doi:10.3847/0004-637X/822/2/86.
- Moyer, Michael (31 January 2014). "Faraway Planets May Be Far Better for Life". Scientific American. Archived from the original on 28 February 2021. Retrieved 20 April 2015.
- France, Kevin; Duvvuri, Girish; Egan, Hillary; Koskinen, Tommi; Wilson, David J.; Youngblood, Allison; Froning, Cynthia; Brown, Alexander; Alvarado-Gomez, Julian D.; Berta-Thompson, Zachary K.; Drake, Jeremy J.; Garraffo, Cecilia; Kaltenegger, Adam F.; Linsky, Jeffrey L.; Loyde, R.O. Parke; Mauas, Pablo J. D.; Miguel, Yamilia; Pineda, J. Sebastian; Rugheimer, Sarah; Scheneider, P. Christian; Tian, Feng; Vieytes, Mariela (30 October 2020). "The High-energy Radiation Environment around a 10 Gyr M Dwarf: Habitable at Last?". The Astronomical Journal. 160 (5): 237. arXiv:2009.01259. Bibcode:2020AJ....160..237F. doi:10.3847/1538-3881/abb465.
- Cuntz, M.; Guinan, E. F. (10 August 2016). "About Exobiology: The Case for Dwarf K Stars". The Astrophysical Journal. 827 (1): 79. arXiv:1606.09580. Bibcode:2016ApJ...827...79C. doi:10.3847/0004-637X/827/1/79.
- NExScI (27 September 2018). "Purpose of Kepler Objects of Interest (KOI) Activity Tables - Cumulative Table". NASA Exoplanet Archive. Archived from the original on 8 February 2024. Retrieved 7 February 2024.
- Cullum, J.; Stevens, D.; Joshi, M. (7 August 2014). "The Importance of Planetary Rotation Period for Ocean Heat Transport". Astrobiology. 14 (8): 645–650. Bibcode:2014AsBio..14..645C. doi:10.1089/ast.2014.1171. PMC 4126269. PMID 25041658.
- Palma, Chris (n.d.). "The Habitable Zone". ASTRO 801: Planets, Stars, Galaxies, and the Universe. The Pennsylvania State University. Archived from the original on 7 February 2024. Retrieved 6 February 2024.
- Pierrehumbert, Raymond T. (2 December 2010). Principles of Planetary Climate. Cambridge University Press. ISBN 9780521865562. Archived from the original on 12 November 2020. Retrieved 2 September 2015.
- Reynolds, R.T.; McKay, C.P.; Kasting, J.F. (1987). "Europa, tidally heated oceans, and habitable zones around giant planets". Advances in Space Research. 7 (5): 125–132. Bibcode:1987AdSpR...7e.125R. doi:10.1016/0273-1177(87)90364-4. PMID 11538217.
- Riguzzi, F.; Panza, G.; Varga, P.; Doglioni, C. (19 March 2010). "Can Earth's rotation and tidal despinning drive plate tectonics?". Tectonophysics. 484 (1): 60–73. Bibcode:2010Tectp.484...60R. doi:10.1016/j.tecto.2009.06.012.
- Rogers, Leslie A. (2015). "Most 1.6 Earth-radius Planets are Not Rocky". The Astrophysical Journal. 801 (1): 41. arXiv:1407.4457. Bibcode:2015ApJ...801...41R. doi:10.1088/0004-637X/801/1/41.
- Rushby, A.J.; Claire, M.W.; Osborn, H.; Watson, A.J. (2013). "Habitable Zone Lifetimes of Exoplanets around Main Sequence Stars". Astrobiology. 13 (9): 833–849. Bibcode:2013AsBio..13..833R. doi:10.1089/ast.2012.0938. hdl:10023/5071. PMID 24047111.
- Sato, S.; Guerra Olvera, C. M.; Jack, D.; Schröder, K.-P. (25 March 2014). "Habitability around F-type stars". International Journal of Astrobiology. 13 (3): 244–258. arXiv:1312.7431v2. Bibcode:2014IJAsB..13..244S. doi:10.1017/S1473550414000020.
- Schulze-Makuch, Dirk; Heller, Rene; Guinan, Edward (18 September 2020). "In Search for a Planet Better than Earth: Top Contenders for a Superhabitable World". Astrobiology. 20 (12): 1394–1404. Bibcode:2020AsBio..20.1394S. doi:10.1089/ast.2019.2161. PMC 7757576. PMID 32955925.
- Skibba, Ramin (2 October 2023). "What Will Plants Be Like on Alien Worlds?". Wired. Archived from the original on 24 January 2024. Retrieved 24 January 2024.
- Kane, Stephen R.; Barclay, Thomas; Gelino, Dawn M. (30 May 2015). "A Potential Super-Venus in the Kepler-69 System". The Astrophysical Journal. 770 (2) L20: L20. arXiv:1305.2933. doi:10.1088/2041-8205/770/2/L20.
- Tuchow, Noah W.; Wright, Jason T. (1 May 2022). "Potential Habitability as a Stellar Property: Effects of Model Uncertainties and Measurement Precision". The Astrophysical Journal. 930 (1): 78. arXiv:2112.02745. Bibcode:2022ApJ...930...78T. doi:10.3847/1538-4357/ac65ea.
- Walker, J.C.G.; Hays, P.B.; Kasting, J.F. (1981). "A negative feedback mechanism for the long-term stabilization of the earth's surface temperature". Journal of Geophysical Research. 86 (86): 9776–9782. Bibcode:1981JGR....86.9776W. doi:10.1029/JC086iC10p09776.
- Weidner, Carsten; Vink, Jorick (2010). "The masses, and the mass discrepancy of O-type stars". Astronomy & Astrophysics. 524 A98: A98. arXiv:1010.2204. Bibcode:2010A&A...524A..98W. doi:10.1051/0004-6361/201014491.
- Van Der Meer, Douwe G.; Zeebe, Richard E.; van Hinsbergen, Douwe J. J.; Sluijs, Appy; Spakman, Wim; Torsvik, Trond H. (25 March 2014). "Plate tectonic controls on atmospheric CO2 levels since the Triassic". PNAS. 111 (12): 4380–4385. doi:10.1073/pnas.1315657111. PMC 3970481. PMID 24616495.